All published articles of this journal are available on ScienceDirect.
Acoustical Respiratory Monitoring: Historical and Modern Aspects
Abstract
This brief review introduces the reader to some of the various historical and modern methods that are available for the bio-acoustical assessment of patient breathing, with other bio-acoustical processes discussed peripherally. Some simple methods of respiratory assessment of historical interest are first discussed, along with more modern methods of patient acoustical monitoring based on advanced analytic methods.
1. INTRODUCTION
The need for simple yet reliable means of respiratory monitoring has existed since the earliest of times. In antiquity (and even today), respiratory monitoring is relied on careful observation of breathing patterns, focusing especially on rate and rhythm as well as contributions from the diaphragm, thorax (intercostal muscles), and accessory musclesi. The origins of cardiorespiratory patient assessment via acoustical technology begins with the invention of the stethoscopeii by the French physician Rene Theophile Hyacinthe Laënnec (1781-1826) [1]. In his time, auscultation was carried out rather crudely with the physician placing his ear directly on the patient’s chest (Fig. 1). Laënnec, said to be seeking some modesty for a buxom young female patient he was about to examine, instead used a roll made from sheets of paper and placed between his ear and the woman’s chest. This turned out to be a surprisingly effective acoustic interface, and the improvisation soon led to a more formal design based on wood and brass (Fig. 2). Decades later, the traditional binaural stethoscope was developed, followed by a series of technical improvements such as the 1925 radio broadcasting of heart soundsiii or teaching models designed to allow multiple clinicians to listen simultaneously (Fig. 3). Sophisticated electronic systems now exist that offer advanced features such as color spectrographic phonocardiogram analy-sis [2] or digital subtraction phonocardiography [3] (Figs. 4 and 5).
One adjunct to respiratory monitoring that was popular in the past decades but is rarely used today is to tape a wisp of cotton wool to the upper lip so that it is positioned under one of the nostrils, and watch it move with each breath (Fig. 6). In the
operating room in decades past, before advanced monitors became available, continuous respiratory monitoring was also achieved using an innovative arrangement such as that shown in (Fig. 7).
In contemporary medicine, the need for respiratory monitoring has become especially important with the heavy use of opioids and other respiratory depressants during perio-perative care. Despite this clear clinical need, no simple and highly dependable method of continuous respiratory moni-toring has come into routine clinical use, although the Masimo system [4-6] (Fig. 8) and capnography [7-11] come close.
Some methods of respiratory monitoring for unintubated patients are as follows:
(1) Direct observation of chest and abdominal movements.
(2) Nasal/oral capnography.
(3) Nasal/oral thermistor methods (thermistor warms up with expired gases).
(4) Use of respiratory monitoring belts (chest plus abdominal; several subtypes).
(5) Extraction of respiratory information from the photoplethysmograph signal.
(6) Electrical impedance methods (uses a small injected electrical current).
(7) Acoustic methods (e.g., Masimo system for acoustic respiratory rate monitoring (Fig. 8), color spectro-graphic analysis (Fig. 9).
(8) Direct observation of chest and abdominal movements.
For intubated patients, respiratory monitoring is somewhat easier, for example using various implementations of airway.
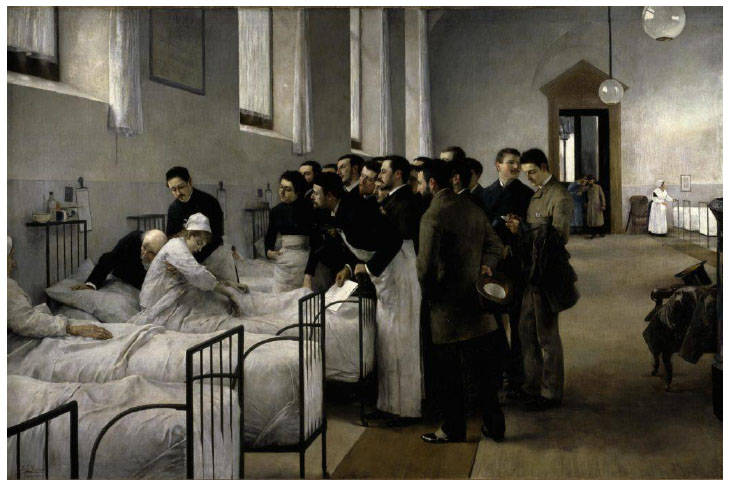
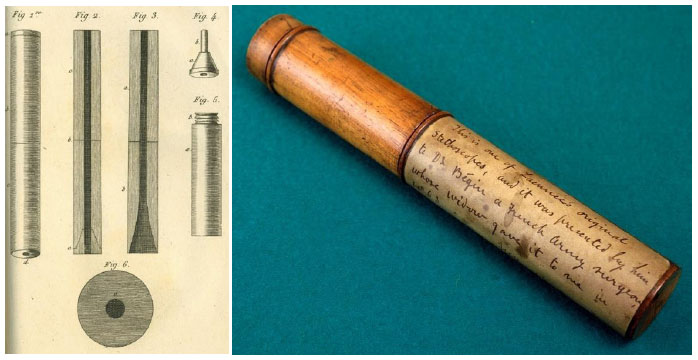
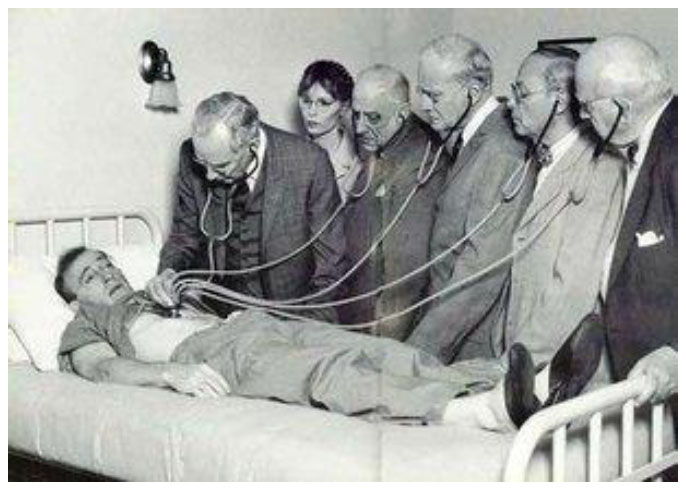
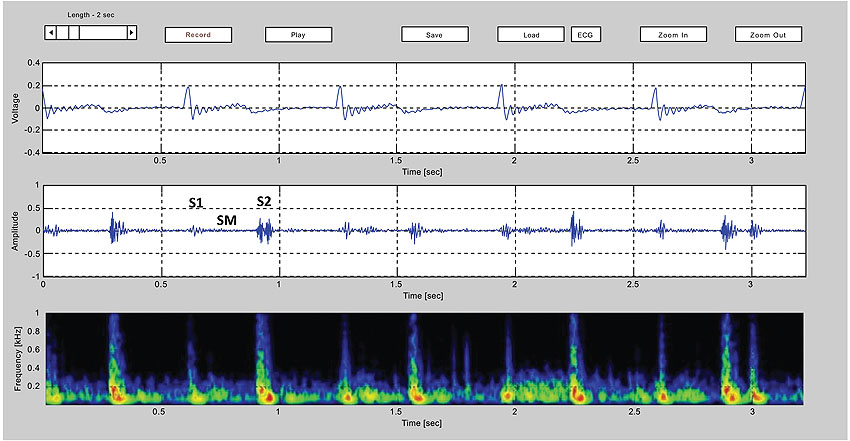
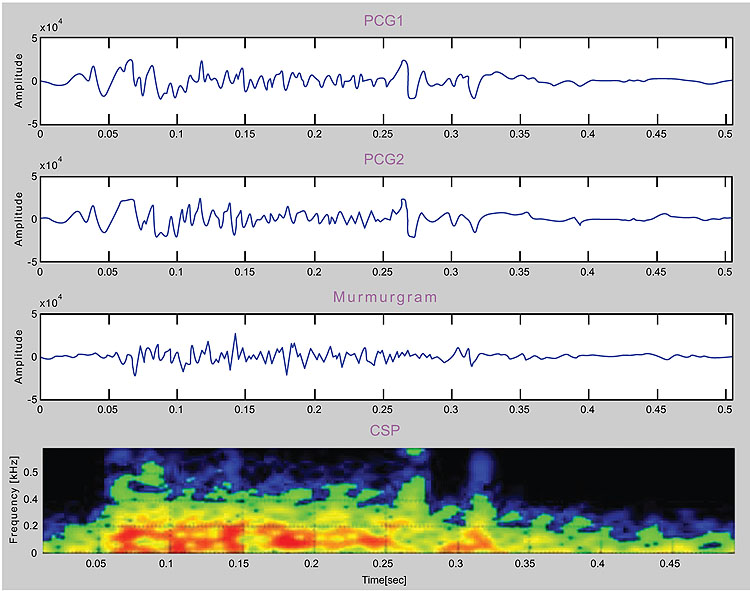
pressure measurement, spirometry or pneumotachography to get quantitative data not ordinarily available to unintubated patients.
Of the methods of respiratory monitoring mentioned above, capnography is amongst the most popular method of continuous respiratory monitoring but suffers from a need to continually ensure that the gas sampling system is operating correctly. Extraction of respiratory information from the pulse oximeter photoplethysmograph signal remains a field of active research [12-19], but has not yet come into the mainstream. A device known as the ExSpiron 1Xi Monitor Pack (Respiratory Motion Inc., Waltham, MA) operates by detecting electrical impedance changes in the thorax and other structures and seems to be particularly promising, but is neither simple nor inexpensive [20-22].
One possible technology for respiratory monitoring that has interested the author for some time is the use of color spectrographic analysis of respiratory sounds. Motivated by the success of color spectrographic techniques in the diagnostic monitoring of rotating machinery [23] and the analysis of bird calls [24], the author set about to build his own system for bio-acoustical research with the goal of developing a research platform for the color spectrographic analysis of respiratory sounds (Fig. 9). The results of these investigations will be reported in future papers.
2. DIRECT AND INDIRECT LISTENING TO BREATH SOUNDS
Precordial stethoscope and esophageal stethoscopes are often used to listen to breath sounds as well as heart sounds, although both are used almost exclusively during surgical anesthesia. Precordial stethoscopes typically employ a weighted chest piece or a conventional stethoscope head in contact with the chest, while esophageal stethoscopes are typically soft, thin-walled tubes inserted into the esophagus inthe unconscious patient. Esophageal stethoscopes usually feature a distal cuff designed to optimize sound transmission and a male Luer connector for attachment to standard ear-pieces. Many models support core body temperature measure-ment as well. Both types of stethoscopes can be very useful in monitoring changes in breath sounds or other bioacoustic phenomena. Examples include the detection of crackles, wheezes and unilateral ventilation resulting from deliberate or inadvertent endobronchial intubation. An excellent detailed review on lung auscultation is available for readers seeking additional information [25].
i For a wonderful video that deals with the various patterns of breathing under ether anesthesia, visit https://www.youtube.com/watch?v=hVY_PTiV90k (The signs and stages of anaesthesia: No. 1 (1944)).
ii The word stethoscope is a combination of two Greek words, stethos (chest) and scopos (examination). But perhaps the word “stethophone” would have been a better choice (see JAMA. 1913;61(25):2260. doi:10.1001/jama.1913.0435026 0058026).
ii For a fascinating account of early (1925) work on heart sound transduction, amplification and transmission by radio, visit https://www.rcplondon.ac.uk/ news/certain-thrill-broadcasting-human-heart
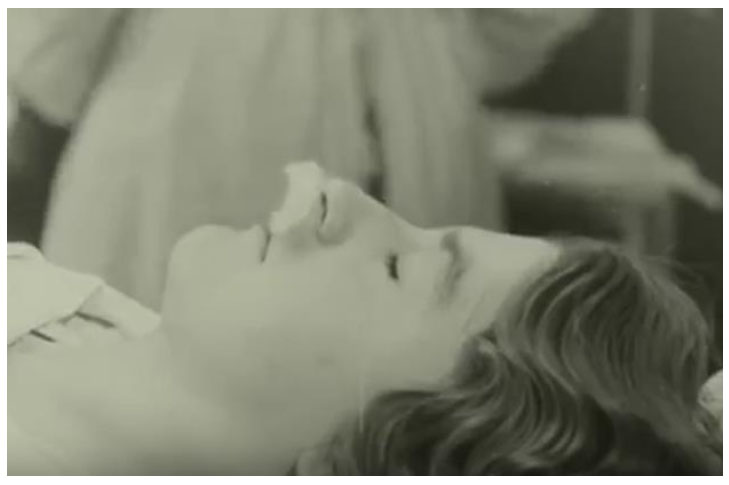
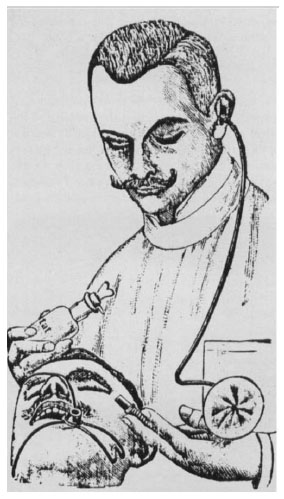
One particularly interesting application of esophageal stethoscopes in pediatric surgery is to access whether the characteristic murmur of a patent ductus arteriosus has vanished following surgical ligation [26, 27]. In another study [28] heart sound intensity was seen to decrease with increasing depth of anesthesia and to increase following the adminis-tration of ephedrine, a cardiac stimulant.
In addition to directly listening to breath sounds via an earpiece, technological innovations have led to developments such as the electronic transduction, amplification and trans-mission of breath sounds as well as in providing methods for advanced computer-based analysis. However, one important aspect central to such undertaking is to evaluate the various stethoscope assemblies to which a microphone is to be attached. I propose to discuss this matter and other technical matters in future papers.
CONCLUSION
Although a variety of methods are available for the assessment of patient breathing, bio-acoustical methods of respiratory assessment offer some special advantages that make them attractive to the clinical community.
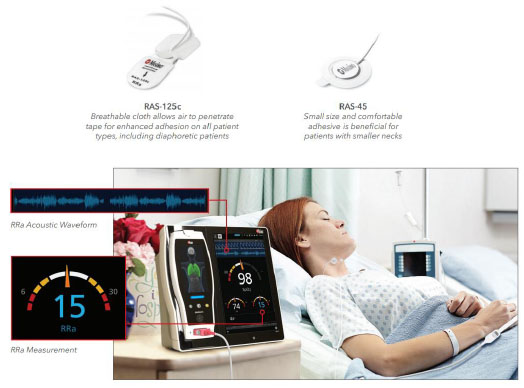
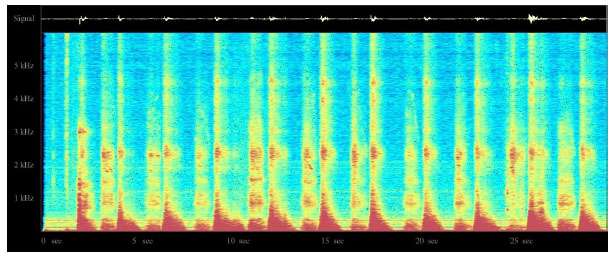
NOTES
i For a wonderful video that deals with the various patterns of breathing under ether anesthesia, visit https://www.youtube.com/watch?v=hVY_PTiV90k (The signs and stages of anaesthesia: No. 1 (1944)).
ii The word stethoscope is a combination of two Greek words, stethos (chest) and scopos (examination). But perhaps the word “stethophone” would have been a better choice (see JAMA. 1913;61(25):2260. doi:10.1001/jama.1913.0435026 0058026).
ii For a fascinating account of early (1925) work on heart sound transduction, amplification and transmission by radio, visit https://www.rcplondon.ac.uk/ news/certain-thrill-broadcasting-human-heart
CONSENT FOR PUBLICATION
Not applicable.
FUNDING
None.
CONFLICT OF INTEREST
The author declares no conflict of interest, financial or otherwise.
ACKNOWLEDGEMENTS
Declared none.