All published articles of this journal are available on ScienceDirect.
Viability of Extracorporeal Membrane Oxygenation Circuits during Arterio-venous Shunting: An Observational Study of ECMO Circuits
Abstract
Introduction
Trial off veno-arterial extracorporeal membrane oxygenation is often performed by diversion of circuit flow through an arterio-venous shunt. Separation from the patient presents an augmented risk for microbiological growth and deterioration of the circuit components and blood circulating within it. It is unclear how long mechanical components and blood circulating within the circuit remain intact during arterio-venous shunting.
Methods
Following decannulation, the arterial and venous lines were connected simulating an arterio-venous shunt. Flow was maintained at 500 ml/min. Observations at 11 intervals were paired with blood culture, blood gases, chemistry and coagulation studies. Heparin was not provided in two circuits, and four circuits received 30 IU/hour.
Results
Of six circuits studied, five maintained flows of 500 ml/min for 48 hours. One circuit developed extensive clot after two hours and blood flow ceased. Microbial growth was not detected. Glucose, fibrinogen and platelet levels reduced progressively from the outset. Cell breakdown was evident by four hours (increase in plasma potassium and phosphate) and lactate rose above 6.3 mmol/L at four hours in all circuits.
Conclusion
Arterio-venous shunting longer than four hours carries a risk of blood decomposition and cell energy pathway depletion. Re-establishing extracorporeal membrane oxygenation support post arterio-venous shunting carries a risk of physiological derangement, given the blood composition changes during arterio-venous shunting. Consideration should be given to an alternate method to assess the physiological stability of patients off extracorporeal membrane oxygenation prior to decannulation. Alternately, consider a crystalloid flush of the circuit to remove decomposed blood if the trial-off exceeds four hours.
1. INTRODUCTION
Extracorporeal membrane oxygenation (ECMO) is a treatment modality for severe cardiac and/or respiratory failure. The indications and decision-making process for weaning from ECMO have been well described [1]. Different approaches have been taken to assess patients off veno-arterial ECMO support prior to decannulation. These include the more commonly used technique of insertion of an arterio-venous (AV) shunt. More recently, pump-controlled retrograde trial-off (PCRTO) has been described as an alternative [1-4].
Insertion of an AV-shunt into the ECMO circuit allows for continued re-circulation of blood whilst support of the patient is temporarily ceased by clamping the cannulae. AV-shunt insertion requires the interruption of patient-circuit continuity, raising concerns over the circuit and blood integrity as in some centres, patients may remain AV-shunted for up to 24 hours [5]. Some centres prevent clotting of the cannulae by intermittently releasing the clamps on the cannulae, allowing intermittent circuit-patient continuity to be re-established, which also avoids blood decomposition in the AV-shunted circuit. Alternatively, if continuous heparin flushes are used for the cannulae, intermittent clamp release may not be necessary.
The optimum duration of AV-shunting remains unknown and there are no guidelines for how long a patient should remain AV-shunted before ECMO support is completely withdrawn. The highly varying patient pathology adds to this lack of consensus. The integrity of an AV-shunted ECMO circuit with regard to blood composition, coagulation profile, microbiological growth and mechanical performance has not been studied. Typically, an assessment of gas exchange capacity and inspection for clots are performed before re-establishing ECMO support following a failed weaning trial. Analysis of blood composition of the circuit prior to re-establishing ECMO support is not routine practice. The duration of safe recirculation before significant blood decomposition occurs remains uncertain. Thus, re-establishing ECMO support with re-circulated blood has the potential to cause significant patient harm. It is also important to understand the progressive breakdown of other cellular components within the AV-shunted circuit and their influence on patient physiology if ECMO flows to the patients are re-established. This study aimed to analyse both circuit and blood integrity during AV-shunting to inform clinicians about the safe duration for AV-shunting during the trial period of ECMO.
2. MATERIAL AND METHODS
We conducted this experimental prospective observational study in a tertiary care paediatric intensive care unit. After weaning and decannulating the patient following an ECMO run, or following circuit change, the ECMO circuit was preserved and modified to simulate a patient undergoing AV-shunting.
2.1. Circuit Design
The ECMO circuit comprised of a centrifugal pump (Rotaflow; Maquet Getinge Group, Rastatt, Germany), albumin-heparin coated diffusion membrane oxygenator (Bioline®, QUADROX-iD pediatric; Maquet Getinge Group, Rastatt, Germany) and 1/4” heparin-free synthetic polymer coated tubing (Softline®, Maquet Getinge Group, Rastatt, Germany). After decannulation, the inlet and outlet lines of the circuit were connected in the sterile field (1/4” x 1/4” connector; Intersept; Medtronic, Minneapolis, USA) to form a re-circulation loop (Fig. 1). The ECMO circuit length was reduced by approximately 100 cm and the haemofilter shunt was removed. A blood storage bag (Fresenius Kabi, Hamburg, Germany) was connected to either side of the newly formed loop via the three-way high-flow stopcocks already in place for routine pressure monitoring. The blood storage bag acted as a reservoir and contained approximately 100 ml of salvaged circuit blood to allow for sampling, thus ensuring no dilutional change in blood composition. A partial clamp was placed on the newly formed arterio-venous loop between the inlet and outlet of the reservoir bag. This ensured that a flow of 250 ml/min was maintained through both the reservoir bag and main loop. A total pump flow of 500 ml/min was maintained throughout the observation period by altering the pump speed. The flow was monitored using a transonic flow probe (System M-M4; Spectrum Medical, Fort Mill, USA). Our centre is a paediatric one, and the majority of our patients are neonates. The blood pump speed during ECMO support is usually set at around 100 ml/kg/min. We set the pump speed at 500 ml/min as this would cover the pump speed usually encountered in a majority of our patients. Pre- and post-oxygenator pressures were measured directly at the oxygenator (pressure display set; DLP, Medtronic, USA).
2.2. Experimental Design
The initial study design entailed stopping the heparin infusion to the circuit at decannulation with no further heparin infusion to the shunted circuit. The first two circuits following this setup showed rapid clotting and/or blood decomposition. The design of the study was therefore amended to include a heparin infusion into the shunted circuit at a rate of 30 IU/hr for the duration of the observation period. The circuit was maintained at normothermia, and gas flow to the membrane was ceased. The circuit was passively monitored throughout the 48-hour observation period. Information was gathered at baseline, 1, 2, 4, 6, 8, 12, 16, 24, 36 and 48 hours. Variables included: RPM, pump flow, pre- and post-membrane pressure and HbSO2 (System M-M4; Spectrum Medical, Fort Mill, USA).
Blood samples were taken for analysis at each of the 11 observation points. Laboratory analysis included haemolytic screen, plasma chemistry studies, coagulation profile, fibrinogen studies, full blood count, activated partial thromboplastin time (aPTT), anti Xa activity, antithrombin and blood culture microbiology. Point of care testing included thromboelastography (TEG® 6s; Haemonetics, Nyon, Switzerland) and blood gas analysis (ABL800 Flex Blood Gas Analyser; Radiometer, Bronshoj, Denmark).
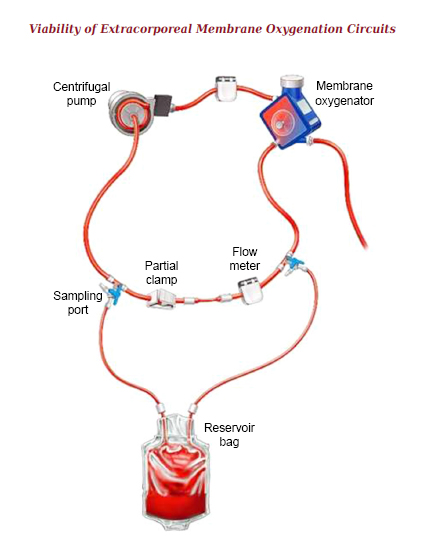
Circuit design simulating AV bridge showing position of sampling port and blood sampling reservoir.
The observation period ceased at 48 hours or when maximum RPM (5,000) was reached and a pump flow of 500 ml/min could not be achieved. In the initial study design, heparin ceased once the experimental circuit was set up and running with the assumption that the heparin effect remained unchanged within the circuit. After the first two experiments, this design was altered, and heparin was infused at a continuous rate for the duration of the observation period.
3. RESULTS
3.1. Demographics and Baseline Physiology
Six circuits from five patients were studied. The median duration of circuit used to provide patient ECMO support prior to the study experiment was 185 hours (range 100 - 500 hours). The circuits studied came from patients who were weaned from ECMO using either PCRTO (veno-arterial patients) or who were weaned from gas flow (veno-venous patients) as previously described [3-5]. The circuits were used for ECMO support in septic, post-cardiac surgical, and respiratory failure patients. The initial plasma haemoglobin, aPTT, platelet count, fibrinogen concentration and trans-membrane pressure (TMP) are given in Table 1.
3.2. Mechanical Observations
Five circuits maintained mechanical performance for the 48-hour study period with a flow of 500 ml/min through the circuit without maximizing RPM. The PaO2 with no fresh gas flow to the membrane was appropriate at baseline (median 156 mmHg; range 74 – 224 mmHg)) and 48 hours (median 138 mmHg; range 128 – 158 mmHg)). Two non-heparinised circuits displayed evidence of clotting within a short time of recirculation. The three circuits observed after the first two non-heparinised ones were heparinised for the time of recirculation and showed preserved mechanical performance of the oxygenator and limited clot formation. One heparinised circuit experienced mechanical failure at two hours. This circuit displayed a rising TMP and significant clot burden in addition to a prolonged period of use (501 hrs) prior to entering the study (Table 1).
The TMP did not increase in three circuits (50%), all of which were heparinised. Increases in TMP were seen in three circuits, two of which were not heparinised. One circuit from the heparinised group displayed a sudden increase in TMP to 400 mmHg at two hours, causing cessation of flow at 5,000 RPM. Both non-heparinised circuits displayed increased TMP to over 150 mmHg; however, flow was maintained throughout the 48-hour study period (Fig. 2).
The RPM of non-heparinised circuits increased by approximately 150% over 48 hours. The RPM of heparinised circuits remained relatively unchanged except for one circuit, which showed a sudden increase to maximum RPM at two hours (Fig. 2).
3.3. Coagulation Studies
There were changes to the coagulation profile in all circuits throughout the observation period. With the exception of the platelet count, there was no difference in values between heparinised and non-heparinised circuits.
Circuit | Heparin Infusion | Support Duration (hrs) | Diagnosis | Support Type | - | Plasma Hb (mg/L) | aPTT (s) | Platelets (x 109 /L) | Fibrinogen (clottable) (g/L) | TMP (mmHg) |
---|---|---|---|---|---|---|---|---|---|---|
1 | No | 161 | Sepsis | VV | - | 703 | 169 | 116 | 1.3 | 14 |
2 | No | 221 | Respiratory Failure | VV | - | 153 | 163 | 65 | 1.6 | 2 |
3 | Yes | 100 | Sepsis | VA | - | 190 | >200 | 75 | 2.6 | 9 |
4 | Yes | 501 | Respiratory Failure | VV | - | 379 | 48 | 104 | 1 | 35 |
5 | Yes | 110 | Post-cardiotomy | VA | - | 223 | >200 | 62 | 1.7 | 9 |
6 | Yes | 209 | Post-cardiotomy | VA | - | 518 | >200 | 64 | 0.5 | 10 |
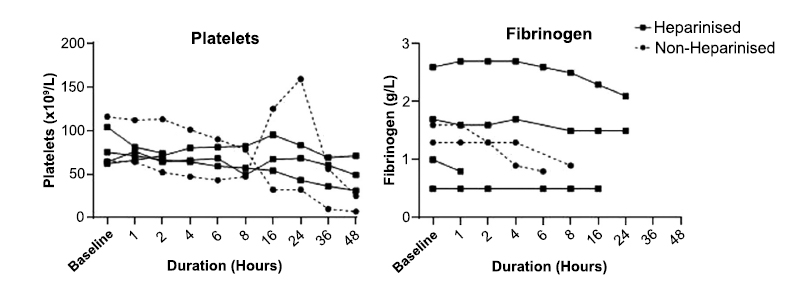
Trans-membrane pressure and revolutions per minute for non-heparinised and heparinised circuits over 48 hours. (TMP: Transmembrane Pressure; RPM: Revolutions per minute).
The activated clotting time (ACT) became prolonged in all circuits, starting at a median of 186 seconds (range 125 – 275 seconds) and increasing to a median of 3,890 seconds (range 527 – 8,030 seconds) at 48 hours. The most substantial increase in ACT occurred between eight and 16 hours, from a median of 242 s (range 153 – 424 seconds) to a median of 836 seconds (range 406 - 4,251 seconds), respectively). The aPTT remained above 80 seconds in all circuits. The heparinised circuits had an aPTT of greater than 200 seconds at all time points.
At baseline, the median platelet count of all circuits was 70 x 109 /L (range 62 - 116 x 109 /L). This decreased to a median of 16 x 109 /L (range 7 - 25 x 109 /L) for non-heparinised circuits and 49 x 109 /L (range 31 - 71 x 109 /L) for heparinised circuits at 48 hours. In one non-heparinised circuit the platelet count peaked at 24 hours, which correlated with an earlier rise and fall in TMP (Figs. 2 & 3). The median fibrinogen of all circuits fell from 1.45 g/L (range 0.5 – 2.6 g/L) at baseline to less than 0.4 g/L at 48 hours in all circuits (Fig. 3). At baseline, the median k time of the citrated RapidTEG™ channel was 2.6 min (range 1.1 – 4.3 min) for all circuits. At eight hours, the median k time was 3.9 min (range .3 – 4.9 min) for all circuits. After 16 hours, the k time was unmeasurable in all but one circuit. At baseline, the median maximum amplitude of the citrated RapidTEG™ channel was 45.3 mm (range 35.6 – 63.3 mm) for all circuits. At 48 hours, median maximum amplitude was 7.1 mm (range 2.1 – 27.1 mm) for all circuits.
The median lactate level for all circuits increased from 2.9 mmol/L (range 2.1 – 3.4 mmol/L) at baseline and more than doubled at four hours. At 48 hours, the non-heparinised circuits had a median lactate of 6.65 mmol/L (range 6.5 – 7.1 mmol/L) compared to the heparinised circuits, which had a median lactate of 12.5 mmol/L (range 10.6 – 14 mmol/L) (Fig. 4).
The median glucose concentration for all circuits decreased from 3.9 mmol/L (range 2.6 – 5.3 mmol/L) at baseline to less than 0.5 mmol/L at six hours in all but one circuit. In one non-heparinised circuit, the glucose level did not fall below 0.5 mmol/L until 16 hours (Fig. 4).
Median potassium level for all circuits increased from 3.7 mmol/L (range 2.9 – 4 mmol/L) at baseline to 25.9 mmol/L (range 22.7 – 32 mmol/L) at 48 hours. Median plasma free Hb at baseline was 301 mg/L (range 153 – 703 mg/L). At 48 hours, non-heparinised circuits had a median plasma free Hb of 34,638 mg/L (range 25,844 – 43,433 mg/L) compared with heparinised circuits which had a median of 18,408 mg/L (range 16,901 - 19,916 mg/L).
Albumin levels remained stable throughout the 48-hour observation period, ranging from 24 g/L to 41 g/L across all circuits. Decreases in white blood cell (WBC) count, neutrophils and lymphocytes were noted, with a decrease for non-heparinised circuits of 6.6 x 109/L, 2.45 x 109/L, 3.27 x 109/L, respectively, over 48 hours. Heparinised circuit WBC count, neutrophils and lymphocytes decreased by 6.8 x 109/L, 1.88 x 109/L and 2.04 x 109/L, respectively, over 48 hours.
4. DISCUSSION
To our knowledge, this study is the first to present observations on circuit integrity and blood composition whilst AV-shunting a patient during a trial-off ECMO. Weaning patients from ECMO remains more an art than a science. Several methods of temporarily separating the patient from the circuit while maintaining blood flow through the oxygenator and remainder of the circuit are used internationally [1]. A trial-off ECMO using temporary patient-circuit separation by AV-shunting the ECMO circuit is a long-established practice. AV-shunting is performed either with indefinite clamping of the patient-circuit connection with concomitant slow heparin-saline flushing of the cannulas or, alternatively, with intermittent release of clamps. The former technique carries greater risk of blood decomposition as the blood continues to circulate independently in an artificial circuit. The latter holds the risk of flushing clots and blood of questionable composition into the patient at repeated intervals during the vulnerable trial-off period.
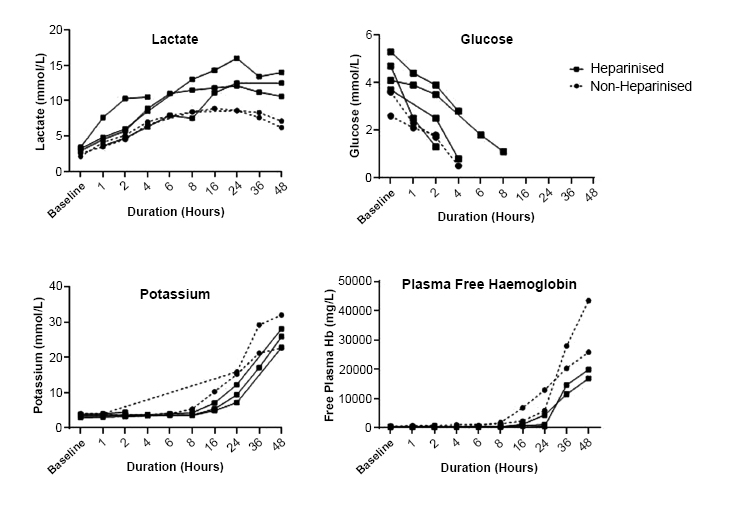
Platelet count and fibrinogen concentration for non-heparinised and heparinised circuits over 48 hours.
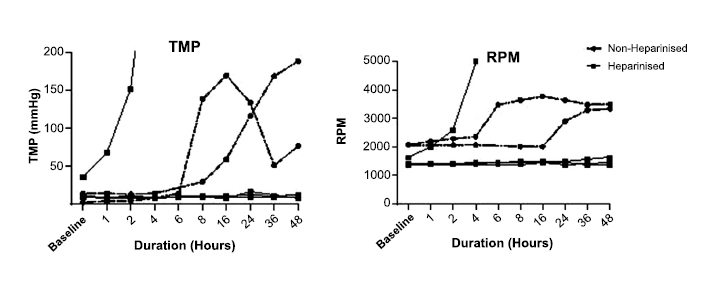
Lactate, glucose, potassium and free haemoglobin concentration for non-heparinised and heparinised circuits over 48 hours.
Our study shows that substantial blood decomposition occurs during AV-shunting. Studies on blood decompo- sition during storage for transfusion purposes have shown that red blood cells (RBC) become depleted of energy sources, leading to a reduced structural integrity of the cell membrane. This leads to the cells becoming less deformable and more fragile, which leads to cell destruction and an increase of plasma haemoglobin, along with an increase in potassium [6]. Breakdown of the cell membrane has also been shown to occur in stored packed red blood cells (PRBC), indicated by a decrease in pH and an increase in potassium and lactate during the initial hours of storage, similar to the observations in our study [6-8]. The breakdown of RBCs can compromise the safety and efficacy of transfusion by reducing the cell’s capacity to carry and release oxygen, and promoting the release of toxic intermediates [9]. We speculate that the cell breakdown described in blood storage is accelerated in an ECMO circuit. Potential causes include circulation through the circuit, continuous agitation of blood and ongoing destruction of red cells by the centrifugal pump. In our study, significant blood decomposition occurred at eight hours, evidenced by a rise in free plasma haemoglobin and potassium. However, a rise in lactate and a reduction in glucose occurred much earlier, which may indicate that cellular metabolism is impaired several hours before cell destruction occurs. Free plasma haemoglobin increased markedly in non-heparinised circuits and to a lesser degree in heparinised circuits, suggesting that the degree of cell destruction was influenced by the presence of heparin (Fig. 4). Free plasma haemoglobin can act as a source of reactive oxygen species and negatively influence physiological rheology either through increased capacity of the RBCs to adhere to the endothelium, or through their enhanced thrombogenic or pro-inflammatory potential, ultimately leading to worse clinical outcomes [8-13]. If the trial-off ECMO using AV-shunting is deemed unsuccessful and the patient is placed back on ECMO support, this “degraded” blood will be rapidly re-infused to the patient. Transfusion of such “degraded” blood has been shown to increase mortality and morbidity in critical illness and could potentially influence outcomes after AV-shunting and re-infusion of “degraded” blood [14-19].
All circuit samples showed coagulation derangements, as evidenced by abnormalities in the TEG, aPTT, fibrinogen and ACT measurements. Some of these abnormalities were induced by heparin which, when continuously infused, improved the mechanical performance of the oxygenator and effectively prevented clot formation. This is in keeping with its primary function when used in extracorporeal systems. Heparin is cleared by two mechanisms: the reticuloendothelial system and endothelial cells, to which heparin binds, as well as renal excretion [20]. However, despite the presence of residual heparin in the AV-shunted circuit, clotting appears to occur within a short span of time without the ongoing addition of heparin. All circuits in the study had evidence of an ongoing heparin effect, though it appears that an aPTT greater than 200 seconds prevents an AV-shunted circuit from clotting. The reasons for this phenomenon remain speculative.
In the clinical setting, AV-shunted circuits are at high risk for circuit thrombosis and continued administration of heparin into the circuit should be considered. However, concerns that infusion into a closed AV-shunted circuit may lead to pressurisation are justified. We postulate that at the infusion rates used in our study, membrane compliance would allow for infusion of such low volumes with minimal risk, especially when AV-shunted for a duration shorter than four hours.
There are no guidelines regarding the safe duration of blood recirculation in an AV-shunted extracorporeal circuit. Current guidelines stipulate that RBCs should be transfused within 4 hours after removal from cold storage [21]. Our data showed that a circuit at room temperature continued to remain free from microbial growth over a 48-hour period. This is similar to data from Brunskill et al. who evaluated current PRBC cold storage guidelines and found that even during prolonged exposure of PRBC to room temperature, there was no microbiological contamination [17].
Our study demonstrates that AV-shunting longer than 4 hours seems to carry the risk of substantial blood decomposition, coagulopathy and cell energy pathway depletion. In comparison to AV-shunting, PCRTO does not carry any theoretical risk of blood decomposition and can be considered safer than AV-shunting in this respect, though this was not tested in our study [3-5]. For patients who will not tolerate PCRTO, it remains unclear whether intermittent unclamping of the AV-shunted circuit could potentially ameliorate the effects of blood decomposition to some extent. In light of the findings in our study, current approaches to the use of AV-shunting for a trial-off ECMO, especially for longer periods of time, merit re-evaluation.
We set the pump speed to 500 ml/min, which is significantly lower than what would be encountered in adult patients. However, we chose such a pump speed given it reflected the majority of the patient population that we support. The flow of 500 ml, however may have influenced our results: a higher blood flow may have resulted in less coagulation activation, but, at the same time, greater cell destruction and blood decomposition.
Limitations of our study are the small sample size. Some centres intermittently clamp (with the intermittent release of clamps) the ECMO circuit during recirculation, thereby “clearing” accumulated lactate and possibly normalising the developing coagulopathy by diluting the circuit blood with patient blood. Our findings would not apply to those scenarios. In addition, our hypotheses should be tested in bigger trials, with a greater variation in blood flow speed, given ours – as mentioned above - was limited to 500 ml/min.
CONCLUSION
Our study suggests that AV-shunting off ECMO for longer than four hours may carry the risk of blood decomposition and cell energy pathway depletion. Substantial blood decomposition may be present even if the circuit appears mechanically intact. Assessment of TMP to evaluate adequacy of pump flow and RPM, along with a visual inspection of an AV-shunted circuit for clot formation may not be adequate to assess circuit safety prior to a decision to re-instate ECMO after a failed trial-off extracorporeal support. A continuous infusion of low dose heparin at low volumes into the circuit may be important to maintain mechanical integrity during the period of AV-shunting.
AUTHORS’ CONTRIBUTION
A. B., A. M., P. V.: Study concept and design; N. S., A. B.: Data collection; N. S., A. B., A. M.: Data interpretation; N. S., A. B., A. M.: Drafting of article; N. S., P. V., N. A., A. M.: Critical revision of the article.
LIST OF ABBREVIATIONS
ACT | = Activated Clotting Time |
aPTT | = Activated Partial Thromboplastin Time |
AV | = Arteriovenous |
ECMO | = Extracorporeal membrane oxygenation |
IU | = International units |
Hr | = Hour |
PCRTO | = Pump controlled retrograde trial off |
RBC | = Red blood cell |
RPM | = Revolutions per minute |
TEG | = Thromboelastogram |
TMP | = Transmembrane pressure |
WBC | = White blood cell |
ETHICS APPROVAL AND CONSENT TO PARTICIPATE
Ethics approval was granted by the local Human Research Ethics Committee (HREC/LNR/19/QCHQ/52188).
HUMAN AND ANIMAL RIGHTS
All human research procedures followed were in accordance with the ethical standards of the committee responsible for human experimentation (institutional and national), and with the Helsinki Declaration of 1975, as revised in 2013.
CONSENT FOR PUBLICATION
Consent was not deemed necessary, given the research only involved circuits.