All published articles of this journal are available on ScienceDirect.
A Simple Acoustic Sensor Test Platform for Respiratory and Cardiac Acoustical Monitoring
Abstract
The properties of various acoustic interfaces (stethoscopes) for respiratory monitoring and phonocardiography are compared using a special-purpose custom-built electronic vibration platform that can be driven by an electronic test signal. Sample sensitivity data for various stethoscope designs are presented in this study.
1. INTRODUCTION
Since the earliest of times, a need has existed for a simple, dependable means of cardiorespiratory monitoring. In antiquity, respiratory monitoring consisted of careful observation of breathing patterns, focusing especially on rate and rhythm as well as contributions from the diaphragm, thorax (intercostal muscles), and accessory muscles. Similarly, in early times, cardiac monitoring primarily focused on the analysis of the pulse, with later innovations in blood pressure measurement and cardiac auscultation contributing in important ways. In modern medicine, the need for respiratory monitoring has become important with the frequent use of opioids and other respiratory depressants, especially during perioperative care or with critically ill patients [1-8]. Given this clear clinical need, the search for simple and dependable methods of patient monitoring has been the focus of numerous researchers, who are looking at acoustical methods of respiratory and cardiac monitoring. To this end, this brief technical report is intended to provide information on building an acoustical test platform of potential value to these investigators.
1.1. Stethoscopes
Precordial stethoscopes and esophageal stethoscopes are often used to listen to breath and heart, especially during surgical anesthesia. Precordial stethoscopes are typically employed on a weighted chest piece or a conventional stethoscope head in contact with the chest (Fig. 1), while esophageal stethoscopes are typically soft, thin-walled tubes inserted into the esophagus in the unconscious patient. Esophageal stethoscopes usually feature a distal cuff designed to optimize sound transmission and a male Luer connector for attachment to standard earpieces. Many models support core body temperature measurements as well. Both types of stethoscopes can be very useful in monitoring changes in breath or other bioacoustic phenomena. Examples include the detection of crackles, wheezes and unilateral ventilation resulting from deliberate or inadvertent endobronchial intubation.
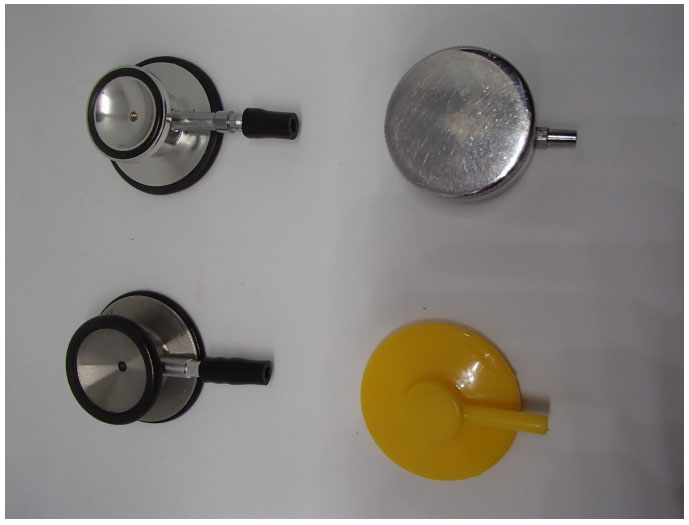
As the science of acoustics progresses, a number of innovations lead to improved stethoscope designs beyond the original 1816 monaural design by René Laennec [9]. For example, important research by Rappaport and Sprague looking at the effects of tubing bore [10] and the quality of the ear fitting [11] lead to innovations in use. In addition to directly listening to breath sounds via an earpiece, further innovations have led to developments, such as the electronic transduction, amplification and transmission of breath sounds, as well as in providing methods for advanced computer-based analysis. However, one important aspect significant to such undertakings is to evaluate various stethoscope assemblies to which a microphone is to be attached. This was the aim of this report.
1.2. Research Platform
While it is relatively easy to calibrate a microphone for use with acoustical respiratory monitoring, it can be somewhat more difficult to compare the effectiveness of various stethoscope assemblies to which a recording microphone is to be attached. As noted, precordial stethoscope assemblies usually come in two forms, the first in the form of a weighted chest piece and the second in the form of a conventional stethoscope head (Fig. 1). In either case, a miniature microphone as shown in the bottom of Fig. (2) is attached to the stethoscope assembly through a short piece of tubing. The question then naturally arises, as to which stethoscope best transfers the vibrations of the connected microphone? This issue was addressed by developing a special test platform for this purpose.
As shown in Figs. (2 and 3) the test platform consists of a small loudspeaker coupled to a plastic vibration test surface and driven by an audio amplifier (located at the bottom right of Fig. 2), this amplifier, in turn, is driven by a signal source such as a function generator. Additionally, the relative sound intensity obtained at the stethoscope under test was measured using a RadioShack 33-3013 miniature omnidirectional electret microphone connected to the stethoscope head through a short piece of tubing and amplified using a second audio amplifier, a RadioShack miniature audio amplifier (Catalog # 2771008) was displayed on a USB oscilloscope (Picoscope 2205).
Table 1 shows the obtained results with a 1000 Hz test signal. Strikingy, there was an approximately 6-fold variation in the sensitivity of the various stethoscope assemblies when tested with a 1000 Hz sinusoid, with the diaphragm of the Littman Classic II SE being the most sensitive (relative sensitivity 1.0) and the bell of the generic model tested type being the least (relative sensitivity 0.16). It is worth noting that different results might be obtained with different stimulus frequencies or conditions where the assembly under test is made to contact the test surface more firmly, for example, using a weight placed on the unit.
2. IDEAS FOR FUTURE WORK
As the objective of this short communication was to introduce the readers of the journal to a simple acoustical test platform that may be of value to investigators, rather than to comparatively evaluate a variety of stethoscopes, the present study investigated the sensitivity of only a limited number of stethoscopes, and only at a single test frequency (1000 Hz). It is hoped, however, that this report will encourage future studies following the footsteps of this initiative.
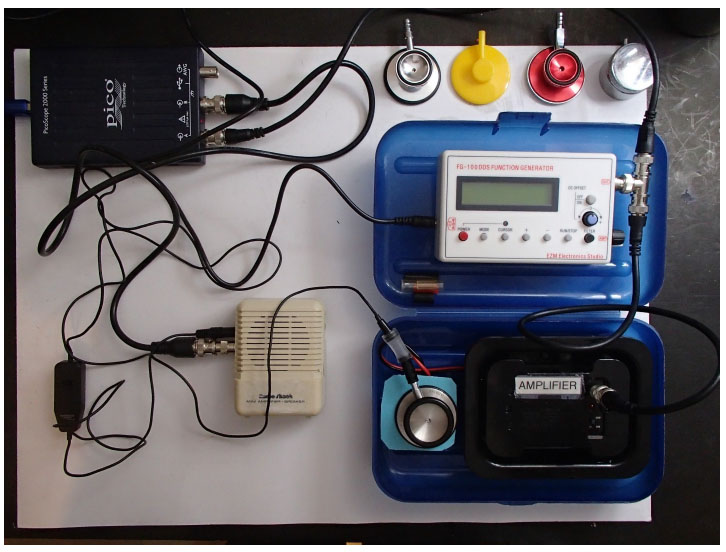
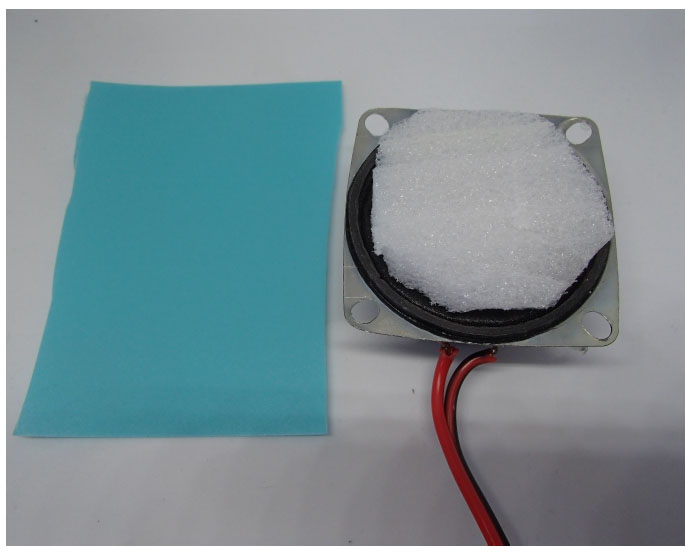
Possible future studies based on the described platform that might be of interest include testing of additional stethoscopes in common use, examining the effect of the degree to which the assembly under test is made to contact the test surface, and (especially) examining the results obtained at frequencies other than 1000 Hz. In the case of the last suggestion, it would be possible to measure the results over a specific range of frequencies using a more advanced setup known as sweep frequency response testing. In such an arrangement, the test signal is made to vary over a range of frequencies (e.g., 50 Hz to 5000 Hz) while the response to the varying test signal is simultaneously recorded using a specialized test apparatus. The result is a frequency response plot (Bode plot), providing a quantitative measure of the spectral characteristics of the system.
Stethoscope Assembly Under Test | Figure Reference | Voltage Ratio |
---|---|---|
Test assembly 1 Littman Classic II SE – diaphragm side |
Figure 1 Bottom left |
1.0 (reference) |
Test assembly 2 Littman Classic II SE – bell side |
Figure 1 Bottom left |
0.55 |
Test assembly 3 Generic stethoscope – diaphragm side |
Figure 1 Upper left |
0.79 |
Test assembly 4 Generic stethoscope – bell side |
Figure 1 Upper left |
0.16 |
Test assembly 5 Diaphragm only disposable stethoscope |
Figure 1 Bottom right |
0.25 |
Test assembly 6 Weighted chest piece |
Figure 1 Upper right |
0.24 |
CONCLUSION
Although a variety of methods are available for the assessment of patient breathing, bio-acoustical methods of respiratory assessment offer some special advantages. To this end, various acoustic interface designs were compared using a special-purpose custom-built electronic vibration platform driven by an electronic test signal. It was found that there was an approximately 6-fold variation in the sensitivity of the various stethoscope assemblies when tested with a 1000 Hz sinusoid, with the diaphragm of the Littman Classic II SE being the most sensitive (relative sensitivity 1.0) and the bell of the generic model tested type being the least (relative sensitivity 0.16).
CONSENT FOR PUBLICATION
Not applicable.
FUNDING
None.
CONFLICT OF INTEREST
The author declares no conflict of interest, financial or otherwise.
ACKNOWLEDGEMENTS
Declared none.